The basic problem
Introduction
Cystic Fibrosis (CF) is a multisystem disease characterised by recurrent lower respiratory tract infections, high sweat chloride, pancreatic insufficiency and male infertility.
Cystic Fibrosis is one of the most common autosomal recessive diseases affecting Caucasians with an incidence between 1:2,000 and 1:90,000, varying between populations. In the UK, the incidence is about 1:2,500 live births and the carrier frequency is 1:25.
With improvement in early diagnosis and treatment, many patients are living into adulthood and middle age. Median survival is around 40 years with median age at death being around 30 years (1). Registry data shows that successive cohorts are progressively living longer and it is anticipated that children born today will live well over 50 years (2). The introductions of effective new therapies which correct the underlying defect are likely to significantly change the long term outlook (3).
Recurrent respiratory tract infections and progressive respiratory failure remains the most common cause of morbidity and mortality in this patient group
Basic genetics
The nucleus of every human cell contains forty-six chromosomes that are made up of long coils of double stranded DNA. Forty-four of these chromosomes are matched into twenty-two pairs and are numbered from 1 to 22 (autosomes). The last pair makes up the sex chromosomes, X or Y. Females receive two X chromosomes while males receive an X chromosome from their mother and a Y from their father.
The chromosomes contain around 35,000 genes. These genes are made up of segments of DNA and are the body’s blueprint for the manufacture of proteins. The latter are the body’s essential building blocks. Each gene comes in as a pair, one inherited from the mother and the other from the father. The exception being those genes on the X chromosome.
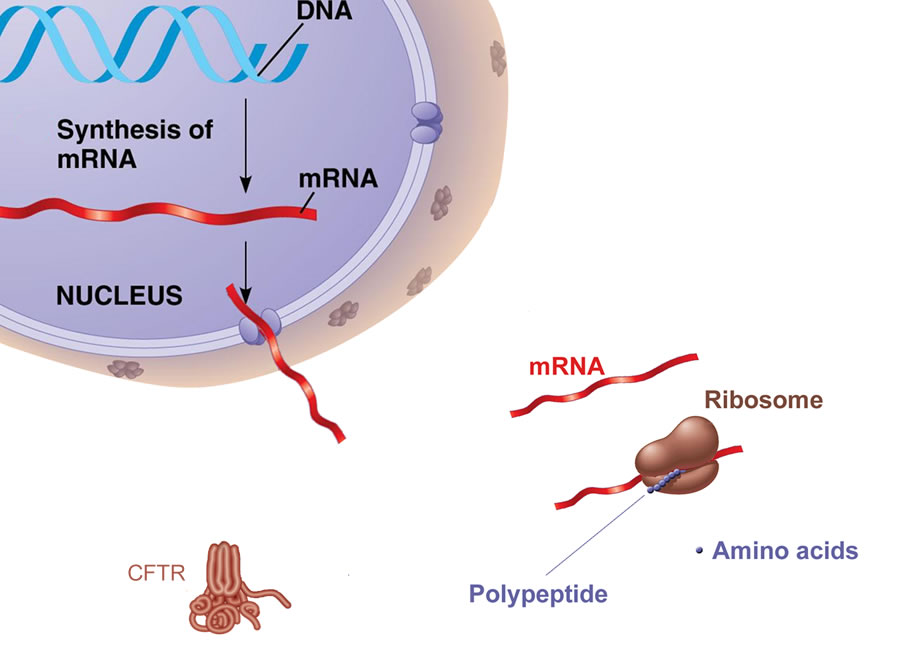
Figure 1. The gene for CFTR is encoded in DNA. In healthy individuals with a normal gene, mRNA produces the 1480 amino acid protein at the Ribosome, The protein is then expressed in the cell membrane throughout the body.
CF mutations
Cystic Fibrosis is the result of genetic mutations (defect) on chromosome 7. The defective gene results in abnormalities in the production and function of a protein called the cystic fibrosis transmembrane conductance regulator (CFTR). The CF gene was localised in 1985 to the long arm of chromosome 7. This followed the identification of the gene sequence in 1989 which encoded a 1480 amino acid protein called the cystic fibrosis transmembrane conductance regulator (4). The first mutation to be identified was p.Phe508del, which occurs in 75% of patients with CF in the UK. This mutation results in abnormal folding, retention in the endoplasmic reticulum and degradation of the CFTR protein. To date over 2000 mutations have been identified with only a handful being responsible for the majority of cases of cystic fibrosis (http://genet.sickkids.on.ca).
Distribution of CFTR
The widespread presence of CFTR throughout the body (lungs, salivary glands, pancreas, liver, kidneys, sweat ducts and reproductive tract) helps to explain why CF is a multisystem condition affecting many organs (figure 2). The two major systems affected are the lungs and the gastrointestinal tract.
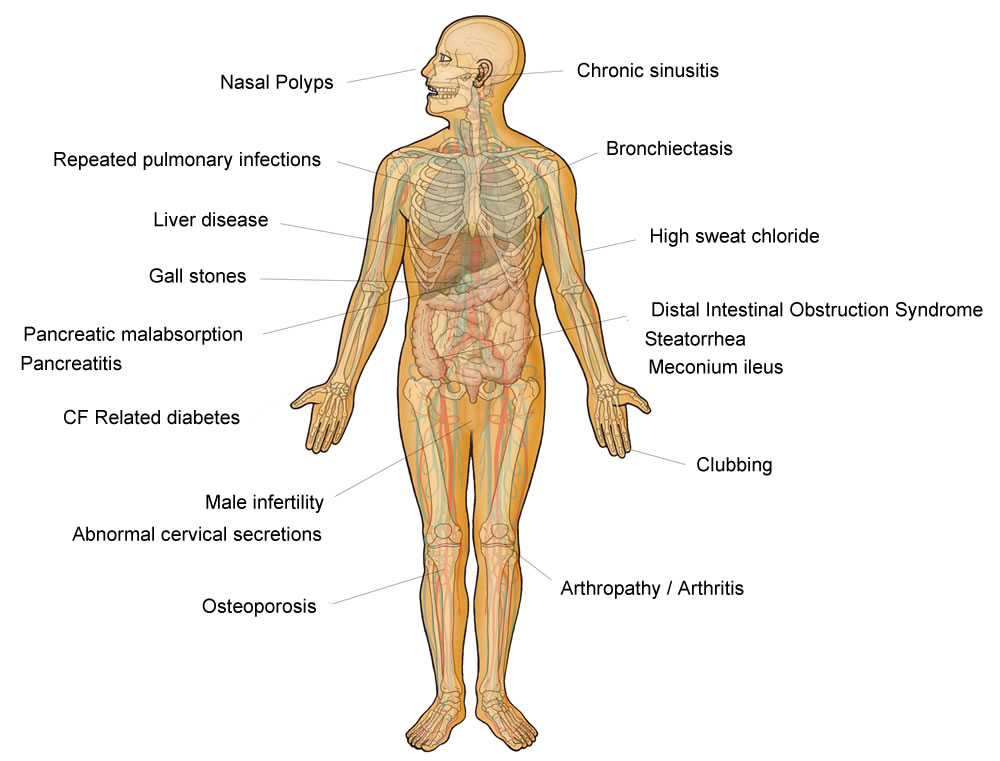
Figure 2. The widespread presence of CFTR throughout the body (lungs, salivary glands, pancreas, liver, kidneys, sweat ducts and reproductive tract) helps to explain why CF is a multisystem condition affecting many organs. The two major systems affected are the lungs and the gastrointestinal tract.
How does CFTR affect the lungs?
In healthy cells CFTR acts as a chloride channel and a regulator of sodium, chloride and bicarbonate transport (5-7). In the lungs, inactive or inefficient functioning CFTR results in impaired chloride transport and enhanced sodium absorption across airway epithelial cells8. This leads to a net increase in water absorption.
The volume of the liquid that sits on the airway surface is reduced and the mucus in the airways becomes more viscid (sticky). In healthy lungs the cilia (small hairs on the surface of the airways) beat in a coordinated fashion so that they continually move mucus up and out of the lungs. This cleansing action of the cilia is impaired in CF because of the presence of very sticky mucus and dry airways (figures 3 & 4) which provide a favourable environment for bacterial infection.
Figure 3. Cilia are fine hair like structures which cover the lining of the airways like a carpet. They beat continuously and act like a conveyor belt to remove bacteria and particles stuck to the mucus layer, driving them from the lungs to the mouth. This cleansing action of the cilia is impaired in CF because of the presence of very sticky mucus and dry airways which provide a favourable environment for bacterial infection.
Structural changes in the CFTR protein have also been linked to defective phagocytosis (ingestion and destruction of bacteria by the white blood cells) such as Pseudomonas aeruginosa, resulting in reduced clearance of infection. Overall these changes in lung physiology lead to dry airways, sticky secretions, a predisposition to chronic chest infections and bronchiectasis (scarring).
Figure 4. In cystic fibrosis, the CFTR protein is defective or absent from the apical membrane. This image shows the normal abnormal transport of chloride ions in healthy and CF airway epithelium
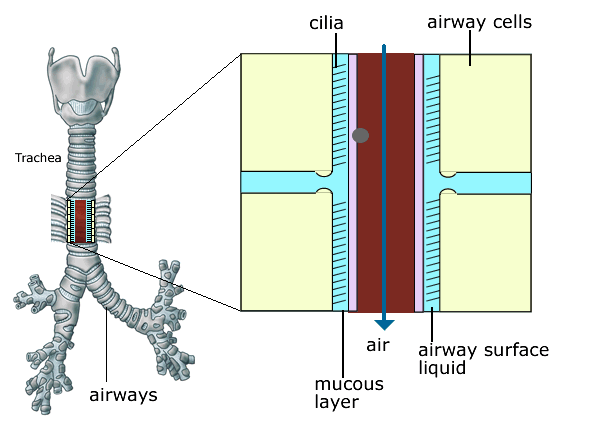
Figure 5 . Normal ciliary clearance of bacteria and foreign particles. Cilia are fine hair like structures which cover the lining of the airways like a carpet. They beat continuously and act like a conveyor belt to remove bacteria and particles stuck to the mucus layer, driving them from the lungs to the mouth.
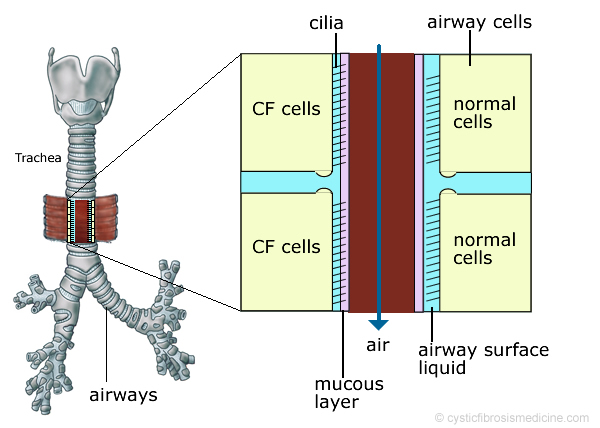
Figure 6. In CF, the production of abnormal secretions and increased fluid absorption across the airway cells leads to drier, stickier secretions. This reduces the normal ciliary clearance of bacteria and foreign particles.
So why do I need treatment if I feel well?
Treatment of CF is aimed at protecting the lungs from pulmonary infections, airway inflammation and permanent lung damage. Individuals with CF may remain relatively asymptomatic despite significant decline in lung function and only develop breathlessness when a critical point is reached and lung reserve is lost. Symptoms are often a poor marker of disease severity, and other factors such as lung function, pseudomonas status and chest radiology should be taken into account (figure 6).
It is important that patients understand this because adherence to medication may be reduced if treatments are perceived as having no immediate impact on clinical status. In some cases drug therapy may transiently increase symptoms in the short term but significantly reduce long term lung damage. For example inhaled antibiotics may initially provoke coughing or some chest tightness and wheezing which settles after a few doses. It is essential that individuals are aware of the natural history of CF and the potential benefits which can result from good adherence and effective prophylactic treatments.
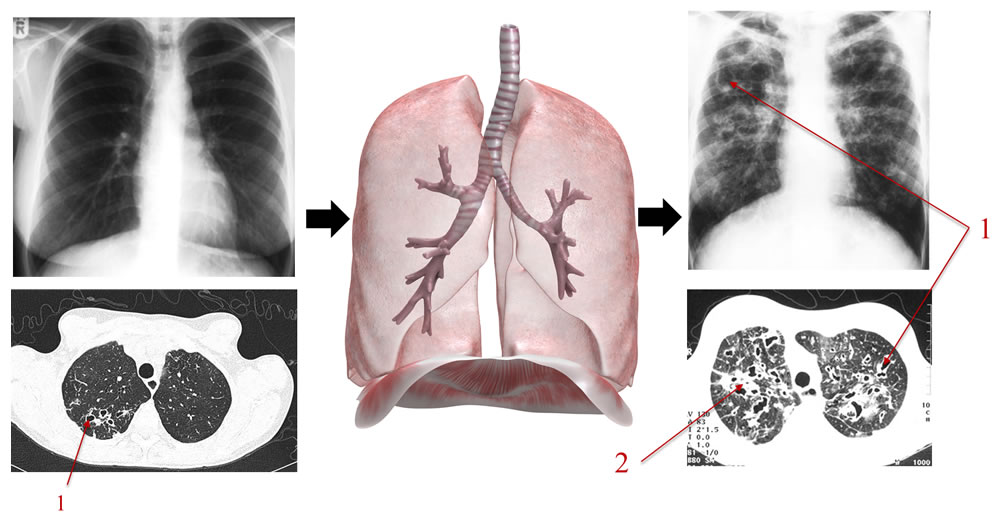
Figure 7. The chest X-ray often underestimates the presence and severity of mild and moderate lung disease. The above diagram shows some of chest X-ray and CT scan changes which oocur in CF (e.g. 1 bronchiectasis/dilated airways, 2 mucus plugging). Treatment aims to prevent/slow down the development of lung damage which can occur insidiously.
Key points
- The CF gene causes an abnormality in the production and function of a protein called the cystic fibrosis transmembrane conductance regulator (CFTR)
- The widespread presence of CFTR throughout the body helps to explain why CF is a multisystem condition affecting many organs
- In the lungs, inactive or inefficient functioning of CFTR lead to drier airways, sticky secretions and a predisposition to chronic chest infections and bronchiectasis (scarring)
- Treatment of CF is aimed at protecting the lungs from pulmonary infections, airway inflammation and permanent lung damage
- Symptoms are often a poor marker of disease severity, and other factors such as lung function, microbiology and chest radiology should be taken into account
References
- Elborn, J. S.; Bell, S. C.; Madge, S. L.; Burgel, P. R.; Castellani, C.; Conway, S.; De Rijcke, K.; Dembski, B.; Drevinek, P.; Heijerman, H. G.; Innes, J. A.; Lindblad, A.; Marshall, B.; Olesen, H. V.; Reimann, A. L.; Sole, A.; Viviani, L.; Wagner, T. O.; Welte, T.; Blasi, F., Report of the European Respiratory Society/European Cystic Fibrosis Society task force on the care of adults with cystic fibrosis. Eur Respir J 2015.
- Dodge, J. A.; Lewis, P. A.; Stanton, M.; Wilsher, J., Cystic fibrosis mortality and survival in the UK: 1947-2003. Eur Respir J 2007, 29 (3), 522-6.
- Ramsey, B. W.; Davies, J.; McElvaney, N. G.; Tullis, E.; Bell, S. C.; Dřevínek, P.; Griese, M.; McKone, E. F.; Wainwright, C. E.; Konstan, M. W.; Moss, R.; Ratjen, F.; Sermet-Gaudelus, I.; Rowe, S. M.; Dong, Q.; Rodriguez, S.; Yen, K.; Ordoñez, C.; Elborn, J. S.; Group, V.-.-S., A CFTR potentiator in patients with cystic fibrosis and the G551D mutation. N Engl J Med 2011, 365 (18), 1663-72.
- Kerem, B.; Rommens, J. M.; Buchanan, J. A.; Markiewicz, D.; Cox, T. K.; Chakravarti, A.; Buchwald, M.; Tsui, L. C., Identification of the cystic fibrosis gene: genetic analysis. Science 1989, 245 (4922), 1073-80.
- Boucher, R. C., Evidence for airway surface dehydration as the initiating event in CF airway disease. J Intern Med 2007, 261 (1), 5-16.
- Borowitz, D., CFTR, bicarbonate, and the pathophysiology of cystic fibrosis. Pediatr Pulmonol 2015, 50 Suppl 40, S24-S30.
- Hwang, T. C.; Kirk, K. L., The CFTR ion channel: gating, regulation, and anion permeation. Cold Spring Harb Perspect Med 2013, 3 (1), a009498.
- Peckham, D.; Holland, E.; Range, S.; Knox, A. J., Na+/K+ ATPase in lower airway epithelium from cystic fibrosis and non-cystic-fibrosis lung. Biochem Biophys Res Commun 1997, 232 (2), 464-8.